Research
Organophosphorus Chemistry
Phosphorus is often referred to as the "carbon copy" because it is isolobal to many common fragments in organic chemistry - including unsaturated, conjugated motifs. Our research focuses on developing the synthesis of novel organophosphorus compounds, and investigating their properties and reactivity.
We have synthesized phosphorus-containing analogues of many simple organic molecules, including cyanide, urea, and isocyanic acid. These organophosphorus compounds provide entry points for the synthesis of more complex phosphorus-containing molecules, and have potential applications for use in other fields such as materials science.
Recently, we have found that a magnesium complex of the cyaphide ion (C≡P–) can be prepared by the reduction of a silyl-functionalized phosphaethynolate. By analogy to Grignard reagents, this can be used to transfer the cyaphide ligand into the coordination sphere of metals through straightforward salt-metathesis reactions.[1a]
PCO– can also be derivatised by reaction with amines under acidic conditions, yielding the corresponding phosphinecarboxamides. These inorganic analogues of urea are rare examples of air-stable primary phosphines, with promising materials applications.[2a]
[1a] D. W. N. Wilson, S. J. Urwin, E. S. Yang, J. M. Goicoechea, J. Am. Chem. Soc. 2021, 143, 10367–10373.
[2a] A. R. Jupp, J. M. Goicoechea, J. Am. Chem. Soc. 2013, 135, 19131–19134
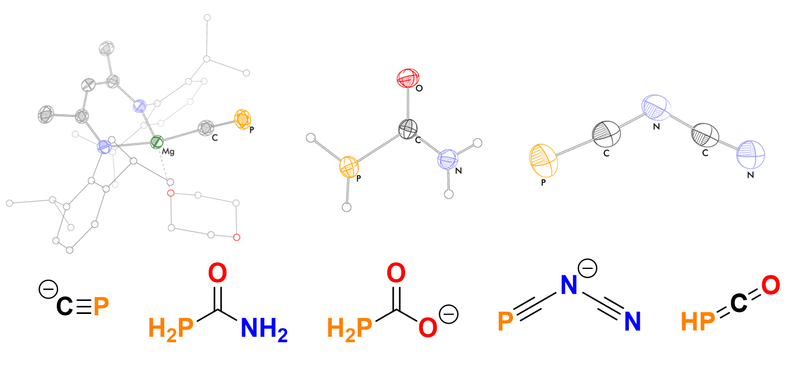
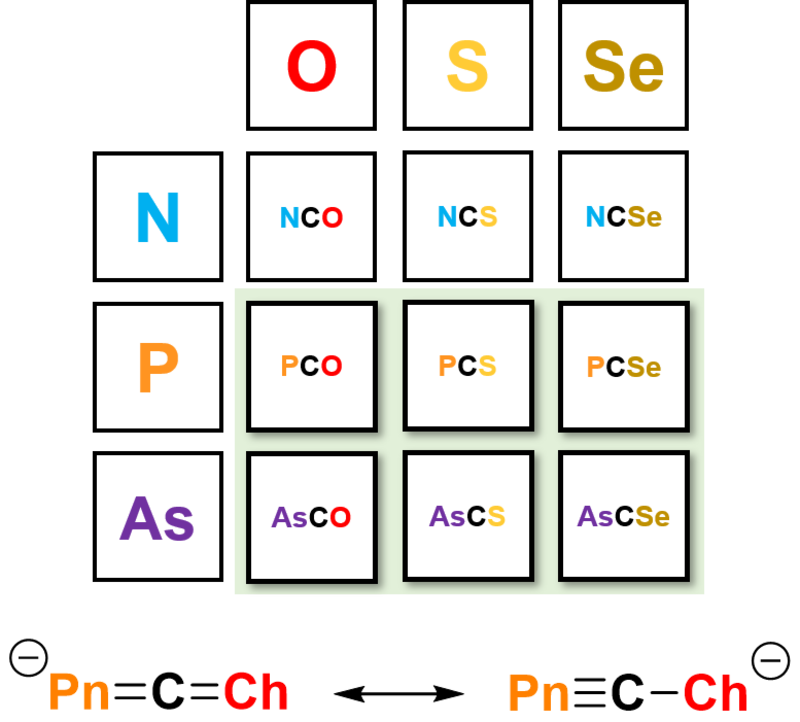
Heavy Cyanate Analogues
The phosphaethynolate anion, PCO–, is a heavy analogue of the valence isoelectronic cyanate ion (OCN−). The phosphaethynolate anion has opened an exciting new avenue of research in the field of organophosphorus chemistry; development over the past 8 years has demonstrated its versatility as a chemical precursor towards, for example, the synthesis of phosphorus-containing heterocycles, as a monoanionic phosphide source, and for low-valent compounds.[1b][2b]
The success of the 2-phosphaethynolate ion prompted us to explore the chemistry of its heavier analogues. The arsenic‐containing ion, AsCO−, was first isolated in 2016 by our research group, and promises to be a similarly versatile reagent in chemical synthesis, having already been employed for the synthesis of several novel molecules.[3b]
We have expanded the series further through a modular approach to the modification of the synthesis. This approach gives access to substitution at both the pnictogen and chalcogen sites, yielding a family of heavy cyanate analogues for further exploration.[4b]
[1b] J. M. Goicoechea, H. Grutzmacher, Angew. Chem. Int. Ed. 2018, 57, 16968-16994
[2b] A. R. Jupp J. M. Goicoechea, Angew. Chem. Int. Ed. 2013, 52, 10064-10067
[3b] A. Hinz, J. M. Goicoechea, Angew. Chem. Int. Ed. 2016, 55, 8536-8541
[4b] F. Tambornino, A. Hinz, R. Koppe, J. M. Goicoechea, Angew. Chem. Int. Ed. 2018, 57, 8230-8234
Multiple Bonds
Multiple bonds involving heavier elements of the p-block are rare due to ineffective p−p orbital overlap, resulting in the tendency for oligomerisation and decomposition.
Recent research has shown that metalla-phosphaketenes, [M]−P=C=O, are capable of undergoing decarbonylation reactions in the presence of a strong nucleophile. We have exploited this strategy for the synthesis of novel compounds with unusual P=E double bonds.
Using this method, we have synthesised the first gallium−phosphorus double bond by using a low valent β-diketiminate gallium (I) nucleophile to induce CO displacement from a phosphanyl-phosphaketene species.[1,2] Additionally, the resulting phosphanyl phosphagallene activates H2 and CO2 in a manner reminiscent of frustrated Lewis pairs.
This opens up the possibility for more examples of E=P double bonds using other low valent nucleophilic main group species. Since arsaketenes are also known, E=As bonds are also likely accessible through this method.
[1] D. W. N. Wilson, J. Feld, J. M. Goicoechea, Angew. Chem. Int. Ed. 2020, 59, 21100.
[2] J. Feld, D. W. N. Wilson, J M. Goicoechea, Angew. Chem. Int. Ed. 2021, ASAP
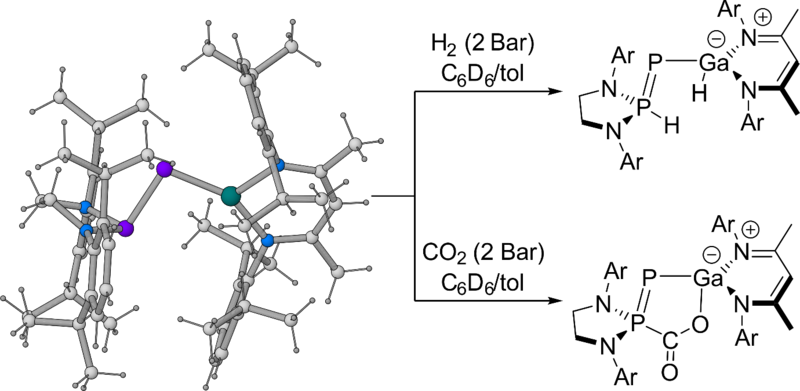
Figure 1. Activation of small molecules by a phosphanyl phosphagallene
Constrained Phosphorus
Oxidative addition processes are often crucial steps in catalytic transformations in transition metal chemistry. These are rare in main group chemistry and play large part in the difficulties with developing main group element-based catalysts. Phosphorus (III) complexes with T-shaped geometries have been observed to undergo formal oxidative addition reactions with small molecules containing H–X bonds.[1] This represents significant progress towards developing inexpensive and environmentally abundant catalysts for chemical processes.
This phenomenon can be explained by a change in electronic structure. The use of tridentate, meridionally coordinating pincer ligands distorts the geometry of the normally trigonal pyramidal phosphorus centre to a more T-shaped geometry. This lifts the degeneracy of the antibonding orbitals whilst not significantly affecting the energy level of the lone-pair. The result is a reduced HOMO/LUMO gap, reminiscent of the electronic structure of other reactive main group compounds such as cyclic alkyl amino carbenes or transition metal d-orbitals.
Recently, we reported phosphorus (III) and arsenic (III) complexes that are able to oxidatively add H–X bonds at room temperature, including NH3 and H2O (Figure 1).[2,3] Ammonia is a notoriously difficult substrate for transition metal-catalysed processes due to the strong tendency to form Werner-type complexes, preventing further functionalisation of the amine ligand. Therefore, main group element-based activation of ammonia is of great interest. These results offer the possibility of metal-ligand co-operative reactivity, or further fine-tuning of the ligand structure for transfer of ammonia to unsaturated organic substrates.
[1] J. Abbenseth, J. M. Goicoechea, Chem. Sci. 2020, 11, 9728.
[2] T. P. Robinson, D. M. De Rosa, S. Aldridge, J. M. Goicoechea, Angew. Chem. Int. Ed. 2015, 54, 13758.
[3] T. P. Robinson, S.-K. Lo, D. De Rosa, S. Aldridge, J. M. Goicoechea, Chem. Eur. J. 2016, 22, 15712.
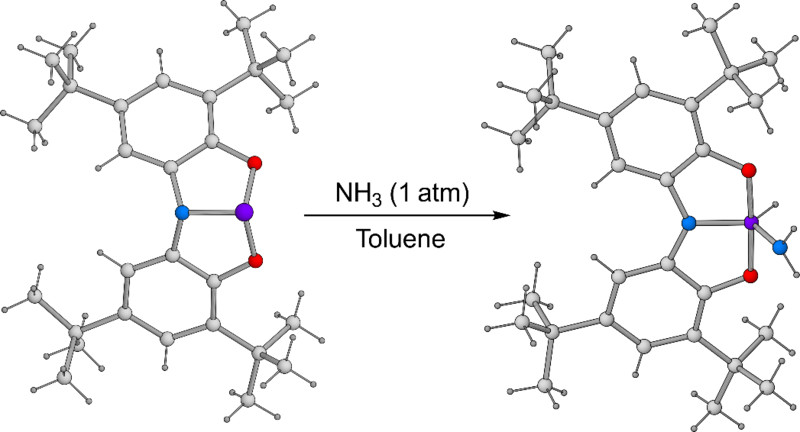
Figure 1. Activation of ammonia using a geometrically constrained phosphorus(III) compound.
Zintl Clusters
Our research group is interested in the solution-phase reactivity of “naked” polyanionic clusters of the group 14 and 15 elements.[1,2] These species can be used as precursors for a wide range of compounds with interesting structures, many of which disobey established rules for bonding. For example, we have pioneered the study of open-shell main group clusters featuring interstitial transition metal atoms, such as [Fe@Ge10]2− and [Ru@Ge12]3− (Figure 1).[3,4] These species represent molecular models of transition-metal/main-group alloys which have found numerous applications in materials science and catalysis.
[1] S. C. Sevov, J. M. Goicoechea, Organometallics 2006, 25, 5678.
[2] R. S. P. Turbervill, J. M. Goicoechea, Chem. Rev. 2014, 114, 10807.
[3] B. Zhou, M. S. Denning, D. L. Kays, J. M. Goicoechea, J. Am. Chem. Soc. 2009, 131, 2802.
[4] G. Espinoza-Quintero, J. C. A. Duckworth, W. K. Myers, J. E. McGrady, J. M. Goicoechea, J. Am. Chem. Soc. 2014, 136, 1210.
Catalysis
More recently, we have focused further into the applications of Zintl clusters towards catalysis. For example, the reaction between [Rh(COD)Cl]2 and K[Ge9{Si(SiMe3)3}3] produces a neutral, alkane soluble cluster, [η4-Ge9{Si(SiMe3)3}3]Rh(COD) (Figure 2), which is the first example of a Zintl cluster homogeneous catalyst, catalysing the hydrogenation or cyclic alkenes at room temperature.[5]
These promising results represent the first steps in a nascent area of chemistry where many interesting breakthroughs await.
[5] O. P. E. Townrow, C. Chung, S. A. Mcgregor, A. S. Weller, J. M. Goicoechea, J. Am. Chem. Soc. 2020, 142, 18330.
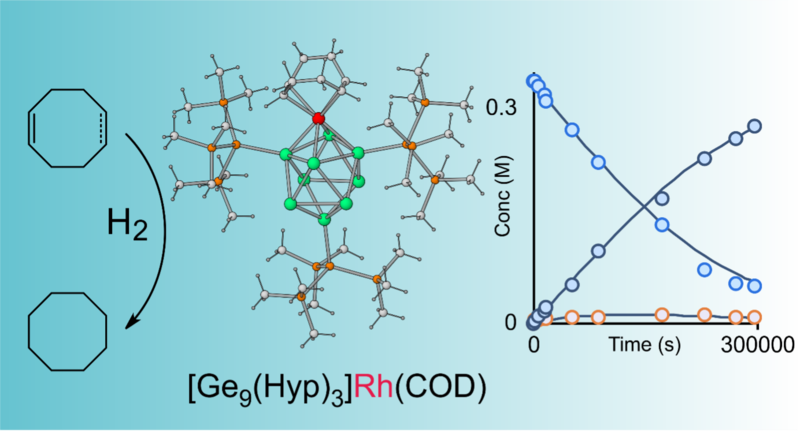
Figure 2. [η4-Ge9{Si(SiMe3)3}3]Rh(COD), the first example of a well-defined Zintl-cluster homogeneous catalyst .